Background
To irrigate apple trees using full/deficit irrigation strategies, estimate yield, and to allocate water correctly, apple trees water needs are to be determined precisely. In 2012, I started a project focused on “detecting water stress, determining water needs, and irrigation scheduling in apple trees”. My research was a contribution to a broader project funded by the National Institute of Food and Agriculture (NIFA). The objectives were to 1) create a site-specific irrigation control and monitoring system in an apple orchard, 2) design and deploy a wireless network of soil, weather and plant sensors, 3) determine water requirements of the trees in real-time, and 4) develop and assess irrigation scheduling algorithms based on main plant-, soil-, and weather-based approaches. The major challenges were a) available ET models like the Penman-Monteith could not explain stomatal regulations in response to a high degree of coupling between apple trees and humidity of the surrounding air, and b) automatic plant-based irrigation scheduling methods had never been used in apple trees and rarely in other tree crops.
I am not going to get into too much detail in this article and will only provide some highlights. Please refer to the publications listed at the end of the article to learn more about my research and modeling efforts.
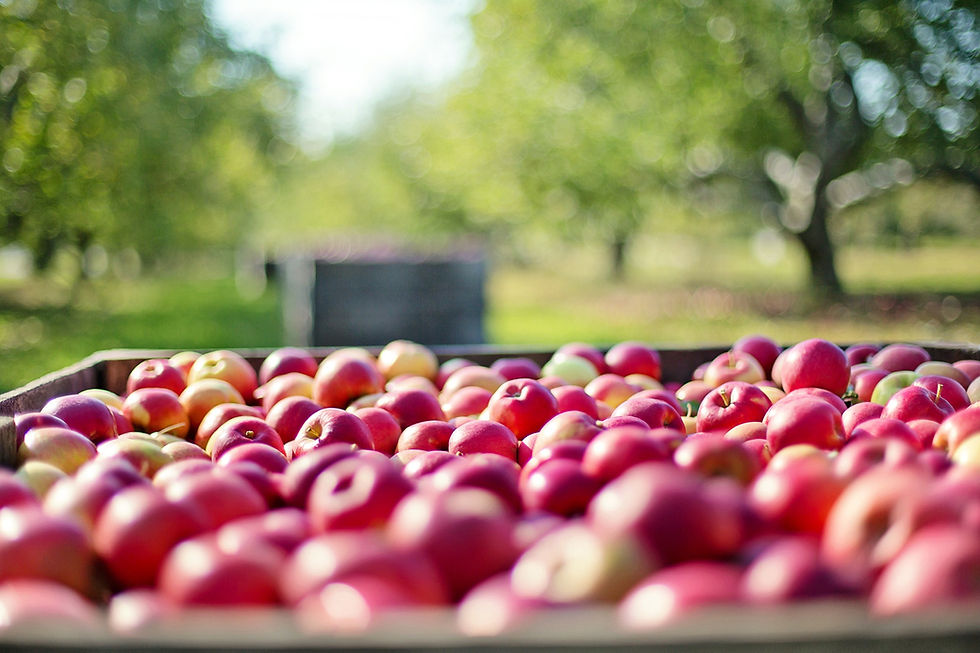
We need to measure ET components in real-time
A few years ago, I came across a comprehensive review of methods available for partitioning between the evaporation and transpiration components of evapotranspiration (ET) estimated by models like the Penman-Monteith (P-M) model (Allen et al., 1998). The conclusion of that study was that future efforts should focus on real-time measurements of evaporation and transpiration, and modeling and simulating ET components separately. There is also a lot of publications on deficiencies of relying on crop coefficient for estimating crop ET. All these studies point at the direction that we need to develop a method for real-time estimation of transpiration that is independent of crop coefficient.
How change in weather parameters can affect plant water needs can be predicted using a transpiration model. The transpiration of apple trees is controlled by a wide range of canopy related factors such as height and density affecting the boundary layer conductance, light intercepted by the trees, and canopy optical/radiative properties determining absorbed radiation. Stomatal conductance, another canopy characteristic, plays a substantial role in water loss from apple leaves.
Apple trees are very different than grass or alfalfa
Current approaches for estimating apple trees water use rely on the P-M ET model. In the single coefficient approach, the evapotranspiration of grass/alfalfa is adjusted by a crop-specific coefficient to calculate crop evapotranspiration. This is while a large number of factors such as various training and irrigation systems, soil management practices, climate, tree age, and cultivar differences make it very difficult to account for everything with only a crop coefficient as described in the FAO-56 method.
Being short and dense, grass/alfalfa canopies have substantial differences with tall, sparse apple canopies. The transpiration of grass/alfalfa is primarily driven by net radiation. Considering a high degree of coupling between apple leaves and the surrounding air, air vapor pressure deficit (VPD) easily affects stomatal activity. Moreover, a large number of factors such as various training and irrigation systems, soil management practices, climate, tree age, and cultivar differences make it very difficult to account for all by a crop coefficient. Maximum values of crop ET in apples are considerably different from year to year.
Besides being affected by water stress, air relative humidity and other environmental factors, it is well-known that the stomatal conductance of apple leaves responds to fruit load. Late in the season after postharvest, lack of fruit load on trees decreases stomatal conductance and consequently actual transpiration which is considerably less than potential transpiration. A similar scenario is also expected when apple trees are in a low-yield year (alternate bearing). This decline in transpiration can challenge a weather-based ET approach which does not account for such a change or reduction in water use. For example, the CropSyst crop model (Stockle et al., 2003) ignores this effect of fruit load on the water use.
Thermal-based ET estimations are better than the P-M
Thermal-based ET estimations are discussed in the literature as an alternative to the P-M approach. Thermal-based ET models are able to sum up the effect of salinity, crop health, and water stress. Canopy temperature measurements are carried out using either thermal cameras or thermal sensors with the latter being less pricey and simpler to use. In canopy temperature monitoring using thermal sensors; however, soil background interference with the readings of thermal sensors or pixels of thermal image lacking enough resolution can lead to significant errors. Considering the sparse nature of apple trees, measurements of canopy temperature is difficult suing a thermal sensor especially during the development stage of tree canopy (early in the season), in which soil background interferes with the readings of the sensor. The estimations of transpiration during this period can be enhanced by introducing an adjustment factor or a plant feedback. If a plant is not transpiring at its potential rate due to any reason including high soil salinity (which makes it difficult for plant to extract water), its temperature increases. This is, at the same time, a challenge for thermal-based models and we need complementary measurements (e.g., soil moisture measurements) to validate thermal measurements.
Actual and potential transpiration models for apple trees
In a number of modeling efforts, we used the energy budget and radiative properties of a single apple leaf as the basis for the development of two theoretical models for estimating potential and actual transpiration of apple trees. The potential transpiration model was based on two main sub-models predicting canopy temperature and total canopy conductance (). The model was derived by simplifying the energy budget of a single apple leaf to rely on only microclimatic data and an empirical coefficient. We also managed to take this study further into developing 1) a novel crop water stress index (CWSI) called Daylight CWSI, and 2) an innovative computer-based irrigation scheduling algorithm named CWSI-DT. Earlier studies revealed that the stomatal activity of apple trees extends beyond midday hours with intense transpiration in the morning and late in the afternoon. Therefore, we hypothesized the suitability of an empirical CWSI averaged over daylight hours for the purpose of continuous monitoring of water status. The new index exhibited high sensitivity to mild variations in the soil water content and stability under transitional weather conditions, suggesting it as a promising indicator of water availability in the root zone. In the latter work, we developed an adaptive irrigation scheduling algorithm relying on a theoretical CWSI to automatically irrigate apple trees. Unlike the traditional CWSI algorithm in which the threshold is a constant value, in the CWSI-DT approach the threshold is dynamically determined by a plant feedback. The results of this study were promising in terms of using ground-based thermal sensing for automatic irrigation scheduling of sparse, discontinuous apple trees.
References
Allen, R.G., Pereira, L.S., Raes, D., Smith, M., 1998. Crop evapotranspiration: guidelines for computing crop water requirements. Irrigation and Drainage Paper No. 56. FAO, Rome, Italy, 300 pp.
Mohamed, A.Z., Osroosh, Y., Peters, R.T., Bates, T., Campbell, C., Ferrer-Alegre, F., 2020. Monitoring water status in apple trees using a sensitive morning crop water stress index. Irrigation and Drainage, 2020: 1–15.
Osroosh, Y. et al., 2019. Detecting fruit surface wetness using a custom-built low-resolution thermal-RGB imager. Computers and Electronics in Agriculture, 157: 509-517.
Osroosh, Y. et al., 2018. Economical thermal-RGB imaging system for monitoring agricultural crops. Computers and Electronics in Agriculture, 147: 34–43.
Osroosh, Y., Peters, R.T., Campbell, C., Zhang, Q., 2016. Comparison of irrigation automation algorithms for drip-irrigated apple trees. Computers and Electronics in Agriculture, 128: 87–99.
Osroosh, Y., Peters, R.T., Campbell, C., 2016. Daylight crop water stress index for continuous monitoring of water status in apple trees. Irrigation Science, 34(3): 209–219.
Osroosh, Y., Peters, R.T., Campbell, C., Zhang, Q., 2015. Automatic irrigation scheduling of apple trees using theoretical crop water stress index with an innovative dynamic threshold. Computers and Electronics in Agriculture, 118: 193–203.
Osroosh, Y., Peters, R.T., Campbell, C., 2015. Estimating potential transpiration of apple trees using theoretical non-water-stressed baselines. Journal of Irrigation and Drainage Engineering, 141(9): 04015009.
Osroosh, Y., Peters, R.T., Campbell, C., 2014. Estimating actual transpiration of apple trees based on infrared thermometry. Journal of Irrigation and Drainage Engineering, 141(8): 04014084.
Stockle, C.O., Donatelli, M., Nelson, R., 2003. CropSyst, a cropping systems simulation model. Eur. J. Agron., 18:289–307.
Comments